Closing the "Summer-Winter-Gap"
Wind, biomass and sector coupling enable a sustainable, efficient and secure energy supply.
24 hours a day, 365 days a year.
EVN's vision for the path to decarbonisation
EVN is a leading, international, listed energy and environmental services company. As such, we want to play an active role in transforming the energy system. We are therefore working intensively on how, when, and by what means a sustainable energy future can be achieved. A data-based approach and analysis of the current situation are essential for developing cost-optimized target trajectories and obtaining a good basis for decision-making. With the support of the zusammEn2040 project team and the extensive expertise of EVN experts, we were able to develop an ambitious vision of the energy system and its path to decarbonization, which is based on the strong expansion of renewable energy sources, especially wind energy, sector coupling, and storage technologies.
One of the key factors for achieving a functioning and fully decarbonized energy system is the issue of seasonal energy shifting. This involves, for example, how electricity from photovoltaic systems (primarily produced in the summer months) can be used as efficiently as possible, or how it can be converted and then utilized in a different form of energy source. One focus in our analysis was how the summer-winter gap can be closed efficiently, i.e. with minimal conversion losses.
Against this background, the following central propositions were developed in the context of the project, which were quantified and confirmed using APG's energy system model:
All results at a glance
Complete decarbonization requires a massive expansion of renewables in Austria. The efficient combination of solar and wind energy can facilitate a more consistent coverage of the electricity demand. Wind power plants are particularly important in the colder winter months (when PV has low generation capacities) because there is no need for additional conversion of the generated energy into other technologies, which saves costs.
The conversion of the energy system requires a great effort and substantial time, material, and personnel resources. Thus we have to get our act together quickly and considerably speed up our efforts, especially in the "simpler" sectors such as heating and mobility.
We have very ambitious targets. An implementation backlog would mean that we have to resort to expensive technologies, such as direct air capture or similar, as makeshift solutions to achieve the set targets. However, this should be avoided at all costs for financial reasons and to save resources.
The increasing volatility of our energy generation with the strong integration of PV and wind power and the decreasing availability of power plants with controllable output, requires an expansion of flexibility options. In this context, sector coupling technologies, such as power-to-heat systems, can facilitate peak load management. Storage technologies such as batteries, water storage, methane storage, and heat storage systems will also play a central role in the energy system of the future. International cooperation will also be essential to optimally balance out the surpluses and shortages in the interconnected regions.
Hydrogen will mainly be used as a raw material for industrial processes.
To make the use of precious and limited biomass as efficient as possible and to bridge the summer-winter gap, the utilization of biomass and biogas should be limited to the winter months under the premise of "no fire in the summer". In the summer, efficient electricity technologies and geothermal energy systems should cover power supply and heating requirements. With this strategy, it would be possible to close the "summer-winter gap" in Austria to a large extent.
Since it can be used in sectors that are difficult to electrify, biogas can make a valuable contribution to decarbonization by replacing fossil natural gas. To maximize the CO2 savings potential, it is necessary to ramp up biogas production in good time.
Locally generated electricity from PV systems is a valuable energy source for supplying private households. However, the surplus feed-in, particularly at noon, requires dramatically high investments in the electricity grid, even though the energy cannot be used by anyone else at this time anyway.
It is better to use the locally generated energy locally; this is possible through flexibilities in the form of charging infrastructure, hot water systems, or storage units. This allows to keep the amount of surplus feed-in to a minimum, which in turn keeps the costs in the system low and ensures that the energy can be utilized to maximum benefit.
Fundamentals for EVN’s vision of the energy system
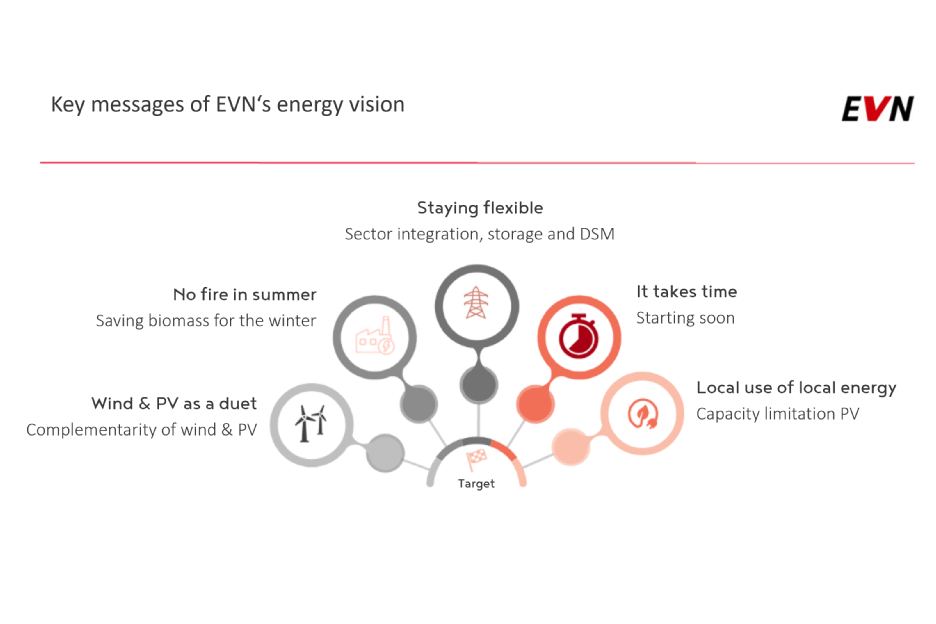
The above-described scenario is only realistic if the right regulatory, economic, and technological framework is developed and implemented. In this context, it is very important to include the general public to create a sustainable change in the energy system.
In our model, we have not specified any parameters regarding changing people's behavior. In our opinion, it is not the job of a public utility company to regulate our customers' lifestyles. Our task is to facilitate the implementation of policies aiming at decarbonizing the economy as a whole by creating suitable conditions and to ensure that in this endeavor EVN's central pillars – security of supply, sustainability, and affordability – are respected without any limitations.
The results of the model-based studies in the context of the zusammEn2040 project in detail
Based on the assumptions made by EVN and the model parameters entered as a result, the energy system model (ESM) calculated the trajectory of the "optimal" energy system until 2050. The most important conclusions are presented below:
Production and consumption of utility-supplied heat via district heating systems
The advantage of biomass is that it is easy to store and is therefore a suitable medium for closing the ‘summer-winter gap’. In a cost-optimized overall system, the use of valuable bioenergy (biomass in heating plants or biomass CHP plants) is shifted to the winter. The correlation is illustrated in Figure 1: In summer, the lower district heating demand in Lower Austria is primarily covered by a combination of power-to-heat plants (heat pumps) and heat storage units. Geothermal energy systems contribute to district heating production all year round. Flexible gas-fired CHP plants and peak load boilers powered by electricity or gas are also needed to secure the district heating supply in times of electricity shortages. The diagram also shows the importance of sector coupling technologies, such as power-to-heat systems, to facilitate the utilization of the electricity generated from renewable sources throughout the year and at peak load times.
Biomass for District Heat Production in Winter Combined with P2H, Geothermal and Power Plants
Production and consumption of district heat
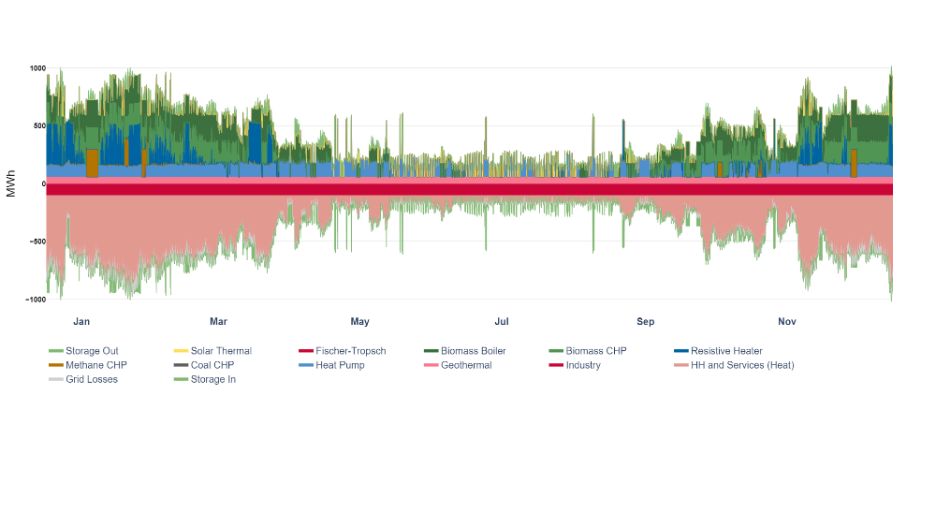
Electricity production and consumption
A massive expansion of renewable production capacities is necessary to cover the sharp rise in electricity demand. The scenario shows that in a European integrated system, a massive expansion of renewable capacities will also be necessary in Austria – if the expansion is facilitated by an appropriate political and regulatory framework, as well as favorable approval procedures: according to the modeling results, 27 GW of wind and 25 GW of PV have to be installed in Austria to achieve complete decarbonization. Lower Austria plays a central role in this context, providing around half of Austria's total wind power capacity. A well-balanced ratio of the two technologies (PV and wind) ensures a cost-optimized coverage of the electricity demand at all times. Pumped storage and run-of-river power plants also continue to play an important role.
A Combination of Wind and PV Power Production Capacities Enhances Cost-Efficient Decarbonisation
PV and wind production capacities in Austria in GW
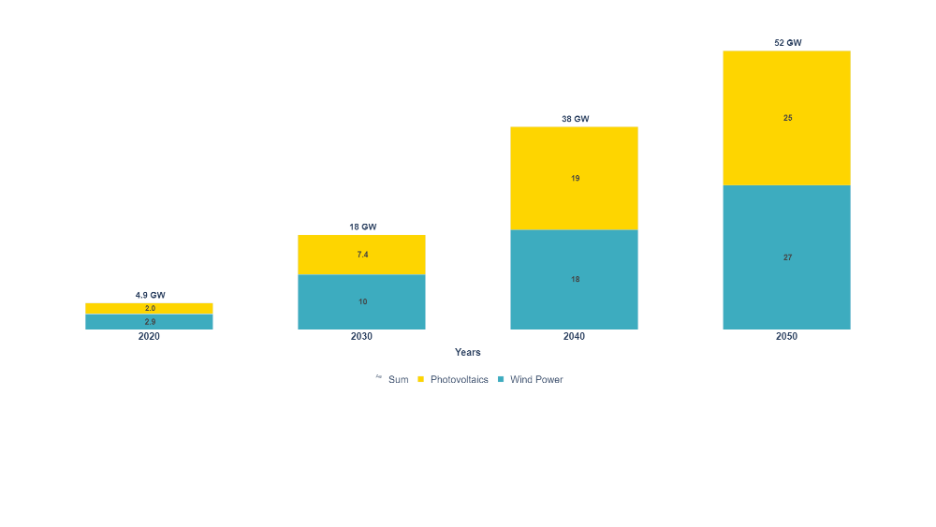
Sector coupling and storage technologies
By modeling the entire value chain from primary production to final consumption, across all steps of conversion and storage, and for all energy sources, the energy system model can show that sector coupling and storage technologies play a central role in efficiently utilizing production surpluses from wind peaks and covering the electricity demand at times of low generation from renewables. Thus it is important to make use of different flexibility options in a way that supports the entire system to utilize the electricity generated from wind in the best possible way. The diagram shows that wind energy can be ideally used for power-to-heat generation, especially in the colder months. Power-to-gas, heat, and electricity storage systems are relevant all year round and make a significant contribution to peak load management.
Some of the electricity generated from renewables (primarily wind energy) is also exported and facilitates pan-European integration. In return, electricity is imported just as frequently, resulting in a largely even balance over the year.
Thanks to the cooperation with neighboring countries and the high flexibility in the utilization of energy, conversion losses can be kept to a minimum, thus creating an efficient and cost-effective overall system.
Electrolysis plays an important role as a sector coupling technology. The decarbonization of the various sectors of the Austrian industry is associated with a high demand for H2 as a raw material. This requires a major expansion and conversion of the current infrastructure.
A Cost-Efficient Mix of Flexibility Options Is Needed To Handle Volatile Wind Power
Comparison of wind power production and usage of flexibility options (P2G, P2H, storages, export) in the decarbonisation year in Austria in MWh power
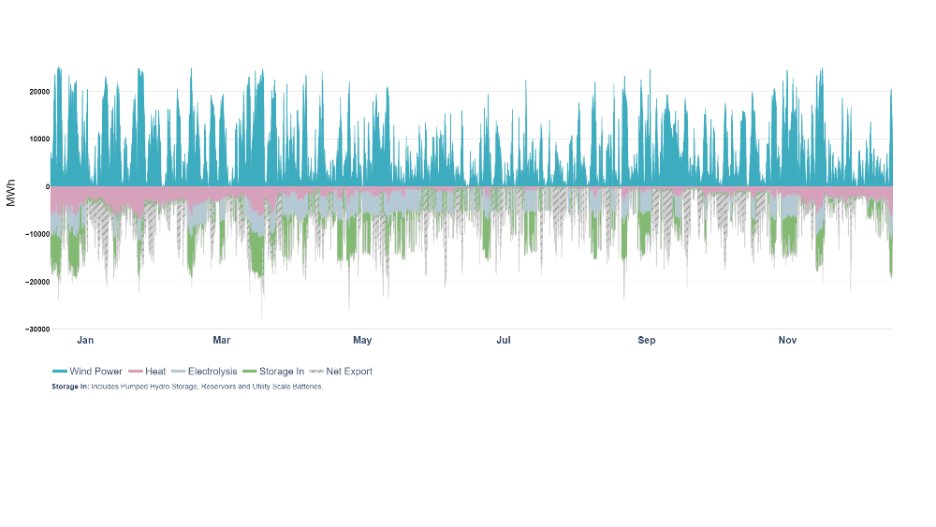
Savings through biogas
Significant amounts of CO2 can be saved by ramping up biogas production capacities in a timely manner. In EVN's modeled vision of the energy system, around 230 TWh of biogas will be produced and used cumulatively in Austria by 2050. This is roughly equivalent to three times the annual consumption of natural gas in 2023 (75 TWh). This amount of biogas will save around 45 Mt of CO2 by 2050 compared to fossil natural gas. In the year of decarbonization, 12 TWh of the non-electrifiable gas demand from industrial processes (approx. 17 TWh) can therefore be covered with locally produced biogas.
Usage of Biogas Contributes to Decarbonisation due to an Early Substituation of Fossil Gas
Development of biogas production in Austria in TWh
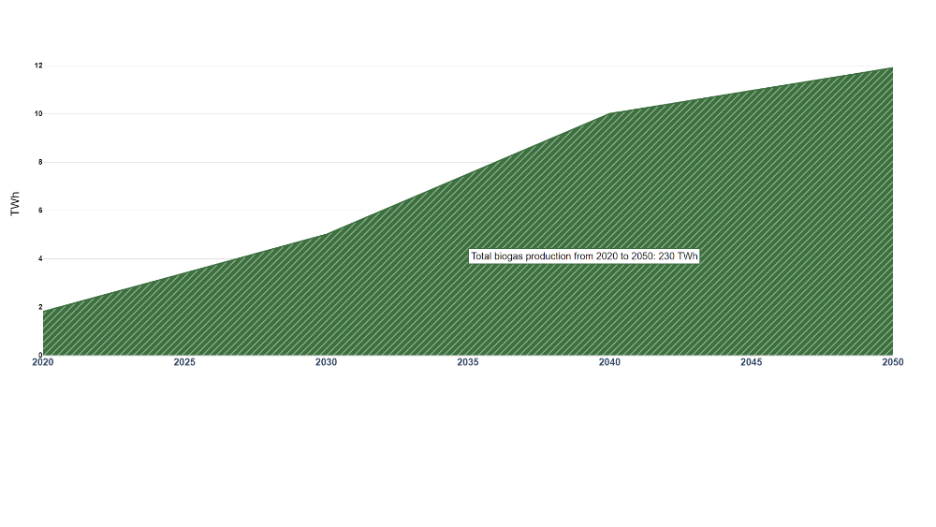
Local use of locally generated electricity
Locally generated electricity from PV systems makes a valuable contribution to the energy future, especially if it is also used locally. This can be optimally ensured with appropriate storage elements in the form of batteries, e-mobility, and hot water systems. This largely avoids the feed-in of production peaks, which cause high grid costs and can hardly be utilized. The diagram below clearly shows that even without an electric car, the feed-in could be limited to around 1/3 of the peak generation without any loss of energy.
Local Usage of Locally Produced Electricity Saves Grid Costs
Example household with 6kWp hot water and PV rooftop with battery on a summer day in kW electricity
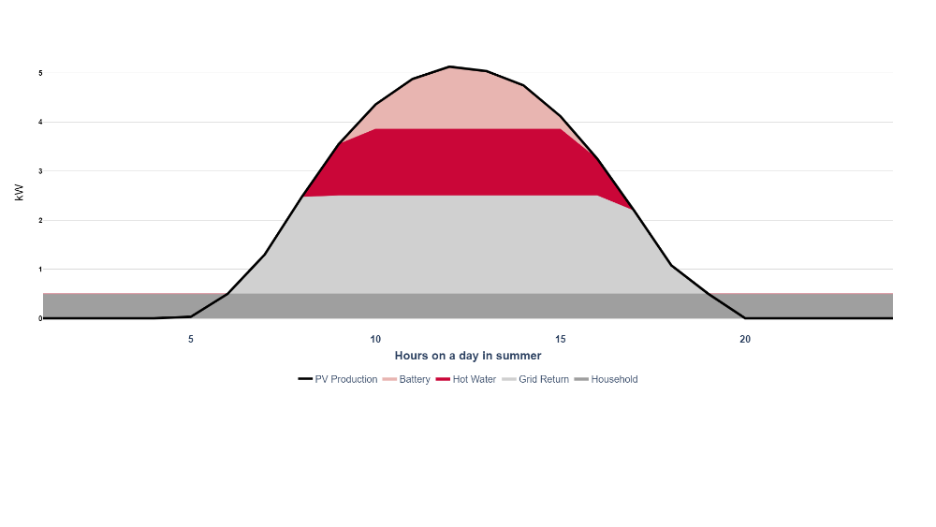
The right regulatory, economic, and technological frameworks need to be developed and implemented to create public awareness concerning the future of the energy system. Clear and efficient approval procedures for different types of technology, incentives such as simple and reality-based electricity tariffs for end customers, and the development of automated and user-friendly DSM tools are just a few examples of where we have identified a great need for action.